
|
|
|
 |
 |
THE CONTEXT FOR S&E INFRASTRUCTURE
HISTORY AND CURRENT STATUS
Today S&E research is carried out in laboratories supported
by government, academe, and industry. Before 1900, however, there
were relatively few government-supported research activities. In
1862 Congress passed the Morrill Act, which made it possible for
the many new states to establish agricultural and technical (land-grant)
colleges for their citizens. Although originally started as technical
colleges, many of them grew, with additional State and Federal aid,
into large public universities with premier research programs.
Before World War II, universities were regarded as peripheral to
the Federal research enterprise. In the years between World War
I and World War II, the immigration of scientists from Europe helped
to develop American superiority in fields such as physics and engineering.
World War II dramatically expanded Federal support for academic
and industrial R&D. The war presented a scientific and engineering
challenge to the United States - to provide weapons based on advanced
concepts and new discoveries that would help defeat the enemy. Large
national laboratories, such as Los Alamos National Laboratory, were
founded in the midst of the war.
The modern research university came of age after World War II when
the Federal Government decided that sustained investments in science
would improve the lives of citizens and the security of the Nation.
The Federal Government increased its support for students in higher
education through programs such as the GI bill. It also established
NSF in 1950 and NASA in 1957. An infusion of Federal funds made
it possible for universities to purchase the increasingly expensive
scientific equipment and advanced instrumentation that were central
to the expansion of both the R&D and teaching functions of the
university.
The advent of the cold war combined with the wartime demonstration
of the significant potential for commercial and military applications
of scientific research led to vast increases in government funding
for R&D in defense-related technologies. The result was a significant
expansion of the R&D facilities of private firms and government
laboratories. Concomitantly, the Federal Government increased its
support for academic research and the infrastructure required to
support it. 11
The U.S. government has been a partner with industry and academe
in creating the S&E infrastructure for many critical new industries,
ranging from agriculture to aircraft to biotechnology to computing
and communications. This infrastructure extends across the Earth's
oceans, throughout its skies, and from pole to pole. Most of the
Nation's academic research infrastructure is now distributed throughout
nearly 700 institutions of higher education; and it extends into
more than 200 Federal laboratories and hundreds of nonprofit research
institutions. Many of these laboratories have traditions of shared
use by researchers and students from the Nation's universities and
colleges. In this role, participating Federal laboratories have
become extensions of the academic research infrastructure.
Assessing the current status of the academic research infrastructure
is a difficult undertaking. Periodic surveys of universities and
colleges attempt to address various aspects of this infrastructure.
But the gaps in the information collected and analyzed leave many
important questions unanswered.
Expenditures for Academic Equipment and Instrumentation
A national survey of academic research instrumentation needs, conducted
nearly a decade ago, provides the latest available information on
annual expenditures for instruments with a total cost of $20,000
or more. As indicated in Table 1, in 1993, the purchase of academic
research instrumentation totaled $1,203 million, an increase of
6 percent over the amount reported in the previous survey in 1988.
The Federal Government provided $624 million, or 52 percent of the
total.
Table 1. 1993 Expenditures for Purchase
of Academic Research Instrumentation of Academic Research
Instrumentation
|
|
$ Millions
|
% Total
|
All Sources of Support |
1, 203
|
100%
|
Federal Sources |
624
|
52%
|
NSF |
213
|
18%
|
NIH |
117
|
10%
|
DoD |
106
|
9%
|
Other Agencies |
186
|
15%
|
|
|
|
Non-Federal Sources |
580
|
48%
|
Academic Institutions |
292
|
24%
|
State Government |
102
|
8%
|
Foundations, Bonds and Private Donations |
105
|
9%
|
Industry |
80
|
7%
|
|
|
|
Source: Academic Research
Instruments: Expenditures 1993, Need 1994, NSF-98-324 |
NSF provided $213 million in support of research infrastructure
during 1993, while NIH provided $117 million and DoD contributed
$106 million. Of the non-federal sources of funding, the largest
single source came from the academic institutions. A sizable contribution
of $105 million came from private, non-profit foundations, gifts,
bonds, and other donations.
A more recent survey of academic R&D expenditures reveals that,
in 1999, slightly more than $1.3 billion in current funds was spent
for academic research equipment. 12
Such expenditures grew at an average annual rate of 4.2 percent
(in constant 1996 dollars) between 1983 and 1999. The share of research
equipment expenditures funded by the Federal Government declined
from 62 percent to 58 percent between 1983 and 1999. In addition,
total annual R&D equipment expenditures as a percentage of total
R&D expenditures were lower in 1999 (5 percent) than it was
in 1983 (6 percent).13
As a point of comparison, during the past decade NSF support of
equipment within a research grant has declined from 6.9 percent
to 4.4 percent of the total grant budget. 14
Capital Research Construction
Biannual surveys of U.S. research-performing colleges and universities
reveal how these institutions fund capital research construction
(costing $100,000 or more), in contrast to research instrumentation.
The Federal Government's contribution to construction funds at the
Nation's research-performing colleges and universities has varied
over the past decade. In 1986-87 it accounted for 6 percent of total
funds for new construction and repair/renovation of research facilities
at public and private universities and colleges. This percentage
increased steadily to 14.1 percent in 1992-93 and then declined
to 8.8 percent in 1996-97. Very recent data indicate this percentage
declined to 6.2 percent in 1998-99. 15
Table 2 indicates that, in 1996-97, research-performing institutions
16 derived
their S&E capital projects funds from three major sources: the
Federal Government, State and local governments, and other institutional
resources (consisting of private donations, institutional funds,
tax-exempt bonds, and other sources).
Table 2. Sources of Funds to Construct and Repair/Renovate S&E
Research Space: 1996-1997
Source of Funds
|
Percent of funds for new construction
|
Percent of funds for repair/renovation
|
|
|
|
Federal Government |
8.7% |
9.1% |
State/Local Government |
31.1% |
25.5% |
Other Institutional Resources |
60.2% |
65.4% |
|
|
|
TOTAL |
100% |
100% |
|
|
|
TOTAL COST |
$3.1 billion |
$1.3 billion |
NOTE: Only projects costing $100,000 or more
SOURCE: National Science Foundation/SRS, 1998 Survey of Scientific
and Engineering Research Facilities at Colleges and Universities.
The Federal Government directly accounted for 8.7 percent of all
new construction funds ($271 million) and 9.1 percent ($121 million)
of all repair/renovation funds. Additionally, some Federal funding
was provided through indirect cost recovery on grants and/or contracts
from the Federal Government. These overhead payments are used to
defray the indirect costs of conducting federally funded research
and are counted as institutional funding.
Another NSF survey representing 580 research-performing institutions
provides some information on the current amount, distribution and
condition of academic research space, which includes laboratories,
facilities, and major equipment costing at least $1 million. As
Table 3 indicates, in 1988 there were 112 million net assignable
square feet (NASF) of S&E research space. By 2001 it had increased
by 38 percent to 155 million NASF.
Doctorate-granting institutions represented 95 percent of the space,
with the top 100 institutions having 71 percent and minority-serving
institutions having 5 percent. In addition, 71 percent of institutions
surveyed reported inadequate research space, while 51 percent reported
a deficit of greater than 25 percent. The greatest deficit was reported
by computer sciences, with only 27 percent of the space reported
as adequate, and more than double the current space required to
make up the perceived deficit. To meet their current research commitments,
the research-performing institutions reported that they needed an
additional 40 million NASF of S&E research space or 27 percent
more than they had.
Table 3. Academic Research Space by S&E Field, 1988-2001
Field |
Net assignable square feet (NASF) in millions
%
|
NASF reported as adequate
|
%
|
% additional NASF needed
|
|
1988
|
1992
|
1996
|
1999
|
2001
|
2001
|
2001
|
All fields |
112
|
122
|
136
|
150
|
155
|
29%
|
27%
|
Agricultural sciences |
18
|
20
|
22
|
25
|
27
|
30%
|
11%
|
Biological sciences |
24
|
28
|
30
|
32
|
33
|
27%
|
32%
|
Computer sciences |
1
|
2
|
2
|
2
|
2
|
27%
|
109%
|
Earth, atmospheric, and ocean sciences |
4
|
7
|
7
|
8
|
8
|
38%
|
26%
|
Engineering |
16
|
18
|
22
|
25
|
26
|
23%
|
26%
|
Medical sciences |
19
|
22
|
25
|
27
|
28
|
23%
|
34%
|
Physical sciences & mathematics |
17
|
17
|
19
|
20
|
20
|
33%
|
25%
|
Psychology & social sciences |
6
|
6
|
7
|
9
|
9
|
32%
|
32%
|
Other sciences |
4
|
2
|
2
|
3
|
3
|
72%
|
18%
|
Note: Components may not add to totals
due to rounding.Source: Survey of Scientific and Engineering
Research Facilities, 2001, NSF/SRS.
|
|
|
|
|
Maintaining the academic research infrastructure in a modern and
effective state over the past decade has been especially challenging
because of the increasing cost to construct and maintain research
facilities and the concomitant expansion of the research enterprise,
with substantially greater numbers of faculty and students engaged
in S&E research. 17
The problem is exacerbated by the recurrent Federal funding of
research below full economic cost, which has made it difficult for
academic institutions to set aside sufficient funds for infrastructure
maintenance and replacement. A recent RAND study estimated that
the true cost of facilities and administration (F&A) for research
projects is about 31 percent of the total Federal grant. Because
of limits placed on Federal F&A rates, the share that the Federal
Government actually pays is between 24 percent and 28 percent. This
share amounts to between $0.7 billion and $1.5 billion in annual
costs that are not reimbursed. Moreover, the infrastructure component
in negotiated F&A rates has increased since the late 1980s,
from under 6 percent in 1988 to almost 9 percent in 1999.18
Unmet Needs
Determining what colleges and universities need for S&E infrastructure
is a difficult and complex task. Nevertheless, over the past decade
a number of diverse studies and reports have charted a growing gap
between the academic research infrastructure that is needed and
the infrastructure provided. For example:
- A 1995 study by the National Science and Technology Council
(NSTC) indicated that the academic research infrastructure in
the U.S. is in need of significant renewal, conservatively estimating
the facilities and instrumentation needed to make up the deficit
at $8.7 billion. 19
- In 1998, an NSF survey estimated costs for deferred capital
projects to construct, repair, or renovate academic research facilities
at $11.4 billion, including $ 7.0 billion to construct new facilities
and $4.4 billion to repair/renovate existing
facilities. 20
- A 2001 report to the Director of NIH estimated that $5.6 billion
was required to address inadequate and/or outdated biomedical
research infrastructure. The report recommended new funds for
NIH facility improvement grants in FY 2002, a Federal loan guarantee
program to support facility construction and renovation, and the
removal of arbitrary caps of the Federal F&A rate.21
- In 2001, the Director of NASA reported a $900 million construction
backlog and said that $2 billion more was needed to revitalize
and modernize research infrastructure. 22
- A recent study indicated that DoE's Office of Science laboratories
and facilities, many of which are operated by universities, are
aging and in disrepair - over 60 percent of the space is more
than 30 years old. A DoE strategic plan identified more than $2
billion of capital investment projects over the next 10 years
(FY 2002 through FY 2011.) 23
- In FY 2001 an informal survey of NSF directorates and the OPP
estimated that future academic S&E infrastructure needs through
2010 would cost an additional $18 billion. 24
- An NSF blue-ribbon advisory panel recently estimated that an
additional $850 million per year in cyberinfrastructure would
be needed to sustain the ongoing revolution in S&E.
25
While these surveys and studies provide a rough measure of the
magnitude of problem, they say little about the cost of lost S&E
opportunities. In a number of critical research fields, the lack
of quality infrastructure is limiting S&E progress. For example:
- The lack of long-term stable support for "wetware"
archives is preventing more rapid advances in post-genomic discoveries.
- The lack of a large-scale network infrastructure that provides
the grounds in which the next generation of secure network protocols
and architectures could be developed and tested will hamper any
significant deployment of these applications.
- The lack of support for new social science surveys, especially
the collection of data in foreign countries, is limiting our scientific
understanding of political events, human opinion and behavior.
- The lack of synchrotron radiation facilities with orders-of-magnitude
increase in luminosity is limiting our ability to extend the frontiers
in such areas as structural biology, genomics, proteomics, materials,
and nanoscience.
THE IMPORTANCE OF PARTNERSHIPS
The international dimensions of research and education are increasingly
essential to U.S. science and engineering. As S&E infrastructure
projects grow in size, cost, and complexity, collaboration and partnerships
are increasingly required to enable them. These partnerships increase
both the quality of the research enterprise and its impact on the
economy and society.
The very nature of the S&E enterprise is global, often requiring
access to geographically dispersed materials, phenomena, and expertise,
as well as collaborative logistical support. It also requires open
and timely communication, sharing, and validation of findings, data,
and data analysis procedures. Projects in areas such as global change,
genomics, astronomy, space exploration, and high-energy physics
have a global reach and often require expertise and resources that
no single country possesses. Further, the increasing cost of large-scale
facilities often requires nations to share the expense.
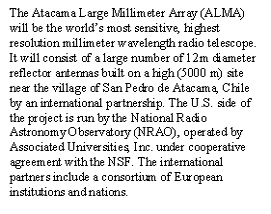
ALMA conceptual image courtesy of the European Southern Observatory
The number of government-funded infrastructure projects that entail
international collaboration has increased steadily over the last
decade. For example, NSF currently supports a substantial and growing
number of projects with international partnering. Among them are
the twin GEMINI Telescopes, the Large Hadron Collider, the IceCube
neutrino observatory at the South Pole, the Laser Interferometer
Gravitational Wave Observatory, the Ocean Drilling Program, and
the Atacama Large Millimeter Array.
In the future, a growing number of large infrastructure projects
will be carried out through international collaborations and partnerships.
The Internet, the World Wide Web, and other large distributed and
networked databases will facilitate this trend by channeling new
technologies, researchers, users, and resources from around the
globe . 26
All large future infrastructure projects should be considered from
the perspective of potential international partnering, or at a minimum
of close cooperation regarding competing national-scale projects.
An additional challenge is maintaining interest in and political
support for long-term international projects. Any absence of follow
through on high-profile projects could increase the danger of the
U.S. becoming known as an unreliable international partner.
Interagency coordination of large infrastructure projects is also
extremely important. For example, successful management of the U.S.
astronomy and astrophysics research enterprise requires close coordination
among NASA, NSF, DoD, DoE and many private and State-supported facilities.
Likewise, implementation of the U.S. polar research program, which
NSF leads, requires the coordination of many Federal agencies and
nations. University access to the facilities of many of the national
laboratories has been facilitated through interagency agreements.
There are a number of models for effective interagency coordination,
such as committees and subcommittees of the White House-led NSTC.
In the fields of high-energy and nuclear physics, NSF and DoE have
developed an effective scheme that facilitates interagency coordination
while simultaneously obtaining outside expert advice. The High Energy
Physics Advisory Panel (HEPAP), supported by NSF and DoE, gives
advice to the agencies on research priorities, funding levels, and
balance, and provides a forum for DoE-NSF joint strategic planning.
This scheme has facilitated joint DoE-NSF infrastructure projects.
For example, the HEPAP-backed plan for U.S. participation in the
European Large Hadron Collider has been credited with making that
arrangement succeed. 27
Partnerships have also played an important role in developing the
genomics infrastructure. For example, the Human Genome Project,
the Arabidopsis Genome Project, and the International Rice Sequencing
Project have made vast amounts of genomic information available
to researchers in the life sciences and other fields. Each of these
projects was accomplished through a strong network of interagency
and international partners.
Partnerships with the private sector also play an important role
in facilitating the construction and operation of S&E infrastructure.
For example, industrial firms have funded much of the equipment
available in the Engineering Research Centers and the National Nanofabrication
Users Network (NNUN). Public-private sector partnerships have also
helped to enable the Internet, the Partnerships for Advanced Computational
Infrastructure (PACI), and the TeraGrid Project.
THE NEXT DIMENSION
While there have been many significant breakthroughs in infrastructure
development over the last decade, nothing has come close to matching
the impact of IT and microelectronics. The rapid advances in IT
have dramatically changed the way S&E information is gathered,
stored, analyzed, presented, and communicated. These changes have
led to a qualitative, as well as quantitative, change in the way
research is performed. Instead of just doing the "old things"
cheaper and faster, innovations in information, sensing, and communications
are creating new, unanticipated activities, analysis, and knowledge.
For example:
- Simulation of detailed physical phenomena - from subatomic
to galactic and all levels in between - is possible; these simulations
reveal new understanding of the world, e.g. protein folding and
shape, weather, and galaxy formation. Databases and simulations
also permit social and behavioral processes research to be conducted
in new ways with greater objectivity and finer granularity than
ever before.
- Researchers used to collect and analyze data from their
own experiments and laboratories. Now, they can share results
in shared archives, such as the protein data bank, and conduct
research that utilizes information from vast networked data resources.
- Automated data analysis procedures of various kinds have
been critical to the rapid development of genomics, climate research,
astronomy, and other areas and will certainly play an even greater
role with accumulation of ever-larger databases.
- Low-cost sensors, nano-sensors, and high-resolution imaging
enable new, detailed data acquisition and analysis across the
sciences and engineering - for environmental research, genomics,
applications for health, and many other areas.
- The development of advanced robotics, including autonomous
underwater vehicles and robotic aircraft, allows data collection
from otherwise inaccessible locations, such as under polar ice.
Advanced instrumentation makes it possible to adapt and revise
a measuring protocol depending on the data being collected.
Research tools and facilities increasingly include digital computing
capabilities. For example, telescopes now produce bits from control
panels rather than photographs. Particle accelerators, gene sequencers,
seismic sensors, and many other modern S&E tools also produce
information bits. As with IT systems generally, these tools depend
heavily on hardware and software.
The exponential growth in computing power, communication bandwidth,
and data storage capacity will continue for the next decade. Currently,
the U.S. Accelerated Strategic Computing Initiative (ASCI) has as
its target the development of machines with 100 teraflop/second
capabilities 28
by 2005. Soon many researchers will be able to work in the "peta"
(1015) range. 29
IT drivers - smaller, cheaper, and faster - will enable researchers
in the near future to:
- Establish shared virtual and augmented reality environments
independent of geographical distances between participants and
the supporting data and computing systems.
- Integrate massive data sets, digital libraries, models,
and analytical tools from many sources.
- Visualize, simulate, and model complex systems such as
living cells and organisms, geological phenomena, and social structures.
With the advent of networking, information, computing, and communications
technologies, the time is approaching where the entire scientific
community will have access to these frontier instruments and infrastructure.
Many applications have been and are being developed that take advantage
of network infrastructure, such as research collaboratories, interactive
distributed simulations, virtual reality platforms, control of remote
instruments, field work and experiments, access to and visualization
of large data sets,30
and distance learning (via connection to infrastructure sites).
31
Advances in computational techniques have already radically altered
the research landscape in many S&E communities. For example,
the biological sciences are undergoing a profound revolution, based
largely on the enormous amount of data resulting from the determination
of complete genomes. Genomics is now pervading all of biology and
is helping to catalyze an integration of biology with other scientific
and engineering fields. In order to fully understand the vast amount
of genomic information available and apply it to improve the environment,
nutritional quality of food, and human and animal health and welfare,
new and improved computational and analytical tools and techniques
must be developed, and the next generation of scientists and engineers
must be trained to use them. Central to genomic sequencing and analysis
is access to high-speed computers to store and analyze the enormous
amount of data. Automated methods for model search, classification,
structure matching, and model estimation and evaluation already
have an essential role in genomics and in other complex, data-intensive
domains, and should come to play a larger role in the future.
The Nation's IT capability has acted like adrenaline to all of
S&E. The next step is to build the most advanced research computing
infrastructure while simultaneously broadening its accessibility.
NSF is presently working toward enabling such a distributed, leading-edge
computational capability. Extraordinary advances in the capacity
for visualization, simulation, data analysis and interpretation,
and robust handling of enormous sets of data are already underway
in the first decade of the 21st century. Computational resources,
both hardware and software, must be sufficiently large, sufficiently
available, and, especially, sufficiently flexible to accommodate
unanticipated scientific and engineering demands and applications
over the next few decades.
|