News Release 13-049
NSF Team Measures Antiproton's Magnetic Charge 680 Times More Precisely Than in Past Efforts
Improvement could help uncover reasons for universe's imbalance between matter and antimatter
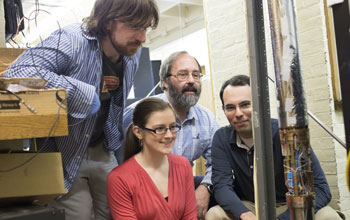
Researchers examine a Penning trap used to measure the magnetic charge of an antiproton.
March 25, 2013
This material is available primarily for archival purposes. Telephone numbers or other contact information may be out of date; please see current contact information at media contacts.
A scientific collaboration that compares hydrogen atoms with their antimatter equivalents has successfully measured the magnetism of a single antiproton to a precision that is 680 times higher than has been possible with earlier methods.
The improvement, reported today in Physical Review Letters, could help scientists uncover why there's a tremendous imbalance between matter and antimatter in the universe.
"A puzzling question about our universe is why it is dominated by matter and there is hardly any antimatter," said Siu Au Lee, physics program director in the National Science Foundation's Mathematical and Physical Sciences directorate, which funded the research. "One way to find out the reason is to probe possible differences between the properties of particles and antiparticles."
"Being able to measure the antiproton's magnetic charge nearly a thousand times more precisely would allow us to see much smaller differences between the size of the antiproton and proton than previously had been possible," added Gerald Gabrielse, the Leverett Professor of Physics at Harvard University, who led the research team.
To accomplish the task, the Antihydrogen trap research team, or ATRAP Collaboration, suspended a single antiproton within a Penning trap at the CERN particle physics laboratory just outside Geneva, Switzerland. The Penning trap used a magnet and a battery to hold the antiproton in a vacuum and sufficiently distant from any matter wall that would cause it to annihilate.
This enabled researchers to see clear evidence that the antiproton was changing the direction of its internal spin when the proton was forced to listen to a radio signal at just the right frequency. The oscillating signal caused the antiproton's internal spin to flip its direction back and forth and allowed researchers to determine the strength of the antiproton's magnetism.
The higher the radio frequency needed to cause the "spin flips," the stronger the antiproton's internal magnet.
The method allowed researchers to compare the strength of the antiproton's magnetism to a proton's magnetism. According to the Standard Model of particle physics--the most fundamental physics theory--the antiparticle (antiproton) and the particle (proton) should have exactly opposite magnets. They should be the same strength, but point in opposite directions with respect to the particle's spin.
In this case, that's precisely what the researchers found-- the antiproton and proton magnets were "exactly opposite" as predicted, even at the new level of precision.
"Precise comparisons of matter and antimatter particles are intriguing in part because the fundamental cause of the asymmetry between antimatter and matter in the universe has yet to be discovered," said Gabrielse. "How does a universe created from equal amounts of antimatter and matter generated in a big bang survive the cooling during which the antimatter and matter would collide and annihilate?"
This is a striking confirmation of one of the more precise predictions of our most fundamental theoretical model, said Gabrielse. "It would have been more fun and given more clues about the universe imbalance if we had observed that the two magnets had had different strengths."
The team believes that the addition of quantum methods will allow even more precise tests in the future.
Assisting Gabrielse with the research were NSF-supported Harvard graduate students Jack DiSciacca, Mason Marshall, Katherine Marable and Rita Kalra, along with postdocs S. Ettenauer and E. Tardiff. Important contributions came from York collaborators Eric Hessels, Cody Storry, Dan Fitzakerley, Matt George and Matt Weel. Jülich Research Center collaborators were Dieter Grzonka, Walter Oelert and Thomas Sefzick.
-NSF-
Media Contacts
Bobbie Mixon, NSF, (703) 292-8485, email: bmixon@nsf.gov
Program Contacts
Siu Au Lee, NSF, (703) 292-7173, email: silee@nsf.gov
Principal Investigators
Gerald Gabrielse, Harvard University, (617) 495-4381, email: gabrielse@physics.harvard.edu
The U.S. National Science Foundation propels the nation forward by advancing fundamental research in all fields of science and engineering. NSF supports research and people by providing facilities, instruments and funding to support their ingenuity and sustain the U.S. as a global leader in research and innovation. With a fiscal year 2023 budget of $9.5 billion, NSF funds reach all 50 states through grants to nearly 2,000 colleges, universities and institutions. Each year, NSF receives more than 40,000 competitive proposals and makes about 11,000 new awards. Those awards include support for cooperative research with industry, Arctic and Antarctic research and operations, and U.S. participation in international scientific efforts.
Connect with us online
NSF website: nsf.gov
NSF News: nsf.gov/news
For News Media: nsf.gov/news/newsroom
Statistics: nsf.gov/statistics/
Awards database: nsf.gov/awardsearch/
Follow us on social
Twitter: twitter.com/NSF
Facebook: facebook.com/US.NSF
Instagram: instagram.com/nsfgov